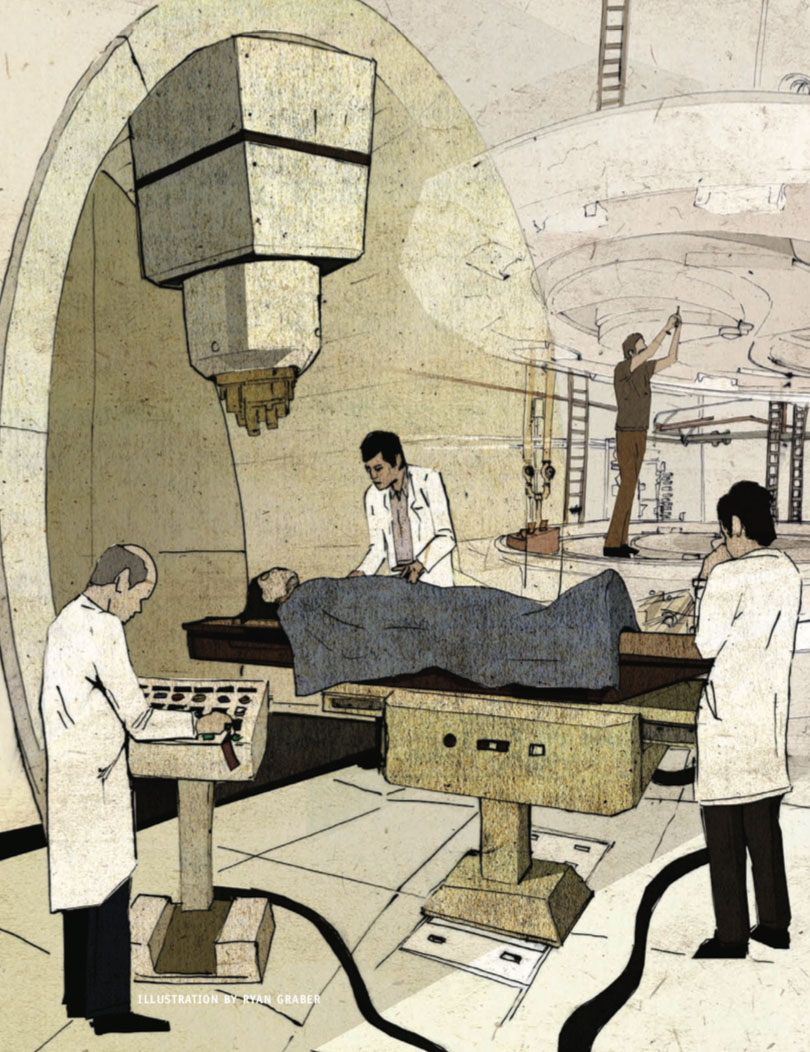
In the last century, American medicine has gone from a cottage industry to a technology-driven juggernaut. The machine at the heart of the new Roberts Proton Therapy Center, dubbed “the world’s most expensive and complex medical device,” provides a glimpse of what the coming years may hold.
By William Hanson | Illustration by Ryan Graber
Sidebar | Countdown to the First Proton-Beam Patient
Sidebar | The Oldest Tool in Medicine
My father was a physician whose career spanned the four decades from the early 1950s to the early 1990s, and many things changed during his lifetime. For example, in the 1950s, the RJ Reynolds Tobacco Company actually ran a campaign with the slogan “More doctors smoke Camels than any other cigarette,” and many of the pictures in my father’s medical school yearbook show him and classmates with cigarettes in hand. However, the really big changes occurred during the latter part of his career (when my own career was just beginning), during which medicine began to evolve from a mom-and-pop, cottage industry into the highly competitive, rapidly advancing, multinational business it is today.
In the late 1970s and early 1980s, I worked at the same medical center that I do now, in an office that was then called Data Processing—the hospital division that managed patient’s bills and accounts payable. There was exactly one computer in the entire hospital—in the basement—and it dined exclusively on IBM punch cards (those heavy, rectangular paper cards formerly used for census-taking, school examination registration, time cards and, perhaps most notoriously, for voting in Florida during the U.S. presidential election in 2000—the piece punched out of the card is, of course, a chad). Punch cards were used to keep track of the medical bills. Data entry clerks punch-typed charges onto the cards, which were then sorted into piles secured with a rubber band. Periodically, the computer would be fed a pile of punch cards upon which it would chew noisily for some time and eventually spit out a ream of data on a teletype machine.
Fewer than 10 people worked in hospital administration—the chief operating officer, the chief financial officer, the chief nursing officer and a few support people. Their job was keep the books, buy things that were needed and make sure the bills got paid—nothing more. The concept of engaging in overt competition to attract patients was inconceivable: The patients just came to us, and the hospital’s officers did pretty much what they were told by the doctors who sat on the hospital’s board.
As I drove to work in the early 1980s, I passed plenty of billboards, none of which advertised hospitals or doctors. In fact, the thought of self-promotion was abhorrent to the medical profession at that point. There were no signs on the city buses asking “Have you been misdiagnosed?” There were very few regulatory agencies, and magazines didn’t publish lists of the best doctors and hospitals. Medical benefits were a little employee perk in the same category as parking and free company business cards.
In short, medicine back then was a pretty sleepy, gentlemanly affair, and some hospitals were like the fat, slow-moving dodos from the island of Mauritius. Today, however, a mere 25 years later, we are in a medical evolutionary arms race. Computer chips are ubiquitous—there are probably 20 in my office alone, what with desktops, laptops, telephones, cell phones, a camera and other gadgets. Data analysts have found their own ecological niche; and 30 percent of health care workers are administrators. In fact, upwards of 30 percent of health care dollars go toward medical administration. Hospitals now have powerful CEOs, many of whom have advanced degrees in health care administration, an educational track that teaches how to control costs, grow product lines and capture market share.
The current landscape of medicine and its place in the larger society is, at best, very confusing when viewed from the ground. We are in what Clausewitz described as the fog of war. On the one hand we hear a steady stream of dire predictions about the all-engorging growth of health care in every modern country in the world, while on the other, there is a competing flow of information about tantalizing new therapies, such as proton beams, that can cure or extend life. Some of the latter will represent major breakthroughs while others will turn out to be no better than or even worse than their less costly predecessors.
Proton beam therapy is a perfect example of the technologies and treatments that are at the leading edge of medicine and that you’ll find in the hospital of the future. Developed by physicists, in their search for fundamental particles, this therapy is technologically sophisticated; even as a critical element of a treatment assembly line, it can be programmed to give treatments precisely calibrated to the specific needs of each individual patient.
Physicists and physicians are both engaged in a seemingly relentless quest to build bigger machines to interact with ever-smaller targets with greater precision; the two fields are inextricably interwoven. Nobel Prize–winning physicist Marie Curie discovered radioactive elements and forms of radiation that are fundamental to the practice of medicine; this same radiation killed both Marie and her equally talented daughter. In 1959, Nobel Prize winner Richard Feynman described nanotechnology and nanoscale medical devices that are now in the process of revolutionizing medicine.
The Higgs boson is the elusive, highly sought after, as yet theoretical fundamental particle that physicists desperately need in order to tidy up one of their theories of how all things work. The boson is the last unobserved member of the particle family belonging to the standard model of physics. Physicists describe it as a rumor crossing a crowded room because it, too, causes transient clustering and massing in the wake of its passage. In essence, the boson is believed to be the fundamental particle that gives all other things—planets, people, and protons—mass.
If a large hadron collider, or LHC, sounds to you like something out of a science fiction movie, you’re not too far off. It is actually the gigantic nuclear particle accelerator and collider located outside of Geneva, Switzerland, at CERN, with which modern physicists plan to find the Higgs boson, using what amounts to an enormous ray gun. The LHC was built with the collaboration of more than 2,000 scientists and hundreds of separate universities and took 10 years to construct. The collider and its associated particle detectors, electron magnets and laboratories are housed in a 26.5-kilometer-long tunnel that is a little less than four meters in diameter and crosses the serpentine border between France and Switzerland several times. It lies at average depths of between 50 and 175 meters underground to minimize its impact on the environment and to prevent harmful radiation exposure to people walking on the land above. The discoveries made within that hidden tunnel will likely change our world above it irreversibly.
This giant machine is designed to fire one beam of protons traveling clockwise at another traveling counterclockwise—both beams at almost the speed of light—in hopes that information about debris from the resulting collision will explain some of the still-unanswered fundamental questions in physics. This a time honored way of finding things out that was invented by and has been practiced for centuries by boys. Fortunately, the proton is a generally well-behaved member of the hadron family (a class of particles composed of quarks), which also includes neutrons and mesons, and the large hadron collider is based on the design of older linear particle accelerators, or atom smashers, upon which, in turn, some of today’s medical radiation devices are modeled.
Over 1,600 superconducting magnets cooled with liquid helium are used to accelerate and steer the proton beams in the LHC. The energy developed in each of the circling proton beams is equivalent to that of a high-speed train traveling at 150 kilometers an hour. When the two beams of protons collide, an unimaginable amount of energy is released—in fact, the temperature at the contact point is a hundred thousand times hotter than the center of the sun. On the other hand, the cooling system for the magnets in the tunnel—the cryogenic distribution system—keeps parts of the collider at temperatures lower than that of outer space.
The protons in the beams make over 11,000 circuits of the tunnel every second, and the attendant forces are so powerful that there is at least a theoretical possibility that the proton collisions will produce microscopic black holes. This sounds really ominous but physicists reassure us that, if formed, these small black holes will deflate, like balloons, by blowing off energy through a process called Hawking radiation, named after the physicist Stephen Hawking.
Proton beam therapy describes the use of a beam of particles, exactly like the ones flying around the 26.5-kilometer hadron racecourse in Switzerland, to treat cancer in humans. Proton beam therapy is only available in a few places in the world. It differs from traditional radiation therapy in its use of beams of particles rather than x-ray waves for treatment. Unlike x-rays that deliver radiation to all the tissues along the path of the beam, causing a little bit of damage all along the way, proton beams pass harmlessly through the skin and overlying tissues to deliver their radiation into the target without injuring the surrounding tissues.
The first suggestion that protons might be used medically to treat tumors came from Robert R. Wilson, one of the physicists who worked on the Manhattan project with Albert Einstein, Richard Feynman and Robert Oppenheimer. A multidimensional scientist like Feynman (who sketched, painted, kept an office in a topless bar and played practical jokes), Wilson was also a sculptor, human rights advocate and a little bit of a rebel. Wilson described the potential for proton therapy in 1946. Having observed that proton beams fired from an accelerator give off a burst of radiation just before they come to a stop, he realized that a beam could be tuned to deliver energy to a very precise area, even one deep within the body.
Wilson proposed that bursts of protons could be trained, like horses, to gallop up to a tumor in formation and then stop on a dime (or on a pinpoint in the case of protons) to deliver their payload of radiation. It is instructive to contrast this elegant medical treatment with what happens in the hadron collider in Switzerland, where two columns of protons are smashed violently into one another at nearly the speed of light, shattering what we once thought were the fundamental particles—neutrons, protons and electrons—into still-smaller particles with names such as charm quark, strange quark, muon, gluon and baryon. In fact, all of the early proton treatments for medical disease were performed in particle accelerators originally built for physics research, such as the one at the Harvard Cyclotron Laboratory, which Wilson helped design, as well as devices in the Soviet Union, Switzerland, Japan and Sweden. The first accelerator built specifically for medical therapy wasn’t constructed until 1988.
Highly targeted proton therapy is particularly useful when a tumor lies in close proximity to critical nerves or organs, such as the brain, prostate, esophagus, lungs and eyes. Because of the proximity of the tumor to its neighbors, the dose of radiation that can be safely administered is limited by the potential for unacceptable injury to critical normal tissue nearby. Proton beams act like smart weapons. The oncologist uses the coordinates of the tumor, based on imaging data from a CT or MRI scan, to design a course of radiation in which the beam is shaped to conform to the silhouette of the lesion. The protons are then energized to the exact level needed to deliver their radiation precisely into the tumor.
I recently had the opportunity to walk around a proton beam accelerator under construction at the Roberts Proton Therapy Center, in the Perelman Center for Advanced Medicine at the Hospital of the University of Pennsylvania. When complete, the center will be part of the largest proton therapy institute in the world, and after having seen the innards of the accelerator before the walls were installed, I can see why this $150 million piece of machinery is called the world’s most expensive and complex medical device. While Geneva’s large hadron collider is the world’s largest scientific instrument, designed to answer big fundamental questions about the universe, its medical counterpart is a worthy little sibling.
The proton therapy cyclotron in the Roberts Center is a 220-ton device at the heart of a football-field-sized building, part of a beautiful medical center designed by the architect Rafael Viñoly. The proton beam generated in the Roberts Center cyclotron can be split into five separate independent sub-beams that are directed to patients by a series of bending, focusing and routing electromagnets. Each of the five beams is delivered into separate suites, multiplying the patient treatment capacity of the center accordingly. The treatment rooms have walls 18 feet thick, like the equipment silos of the hadron tunnel, to contain stray neutron radiation. The building itself, like the CERN collider, is mostly underground, hidden well below the airy superstructure.
At one point during my tour around the scaffolding and curing concrete, I stood at the edge of a doorway, blocked by a flimsy, temporary guardrail, that opened into space halfway up the wall of a cavernous room that will eventually be one of the treatment suites. As I watched, construction workers were welding together a 35-foot high, 90-ton metal cage known as a gantry, capable of rotating 360 degrees around the patient. The proton beam is guided from the cyclotron and then around this gantry, through a nozzle, past the skin and into the cancer with a 20,000-pound magnet; it is shaped just prior to delivery to conform to the exact silhouette of the patient’s tumor. As I stood there with my toes hanging over the sill, looking up one story to the top and down another to the bottom, I was struck by the disproportion between this gigantic machine and the relatively tiny pallet at its center on which the patient will lie during treatment.
When the Roberts Center is finished, that doorway into space will appear to be an ordinary entrance to a functional room and the gantry won’t be visible. Patients will be unaware of the surrounding machinery during a course of treatment because it will be hidden behind the walls. And patients will barely have time to think about what’s happening around them because individual treatments will be short. Still, even a cursory understanding of the events going on during their time there will leave patients with a good measure of shock and awe at the current breadth of our knowledge of physics, human biology and the lengths to which we will go to fix a body when it’s broken.
In order to fully appreciate what an enormous human achievement proton beam therapy represents, it’s instructive to follow what happens in a stepwise fashion between the time hydrogen gas enters the system at one end and the point at which, on our command, a rank of prancing protons comes to a dead stop in the middle of a cancer tumor hundreds of feet away. That tumor may only be a few millimeters large and located in a patient’s eye, such as a two-millimeter melanoma on an otherwise blue iris. Step one is the injection of hydrogen atoms into an electrical field, in which the single electron is stripped off leaving a free, positively charged proton. In the next step, a bunch of these protons are spun around in a cyclotron ten million times a second and are given a little electrical nudge with each rotation, like children on a playground merry-go-round, until they reach an energy level of millions of electron volts. Bursts of these energized, charged particles then exit the cyclotron and are channeled along a virtual proton road to the exact spot that we want them to park, so to speak, to discharge their accumulated energy in a blast and consequently rip electrons right out of the orbits of the molecules making up the cancer cells.
And the protons don’t just tear off the cellular equivalent of a bit of paint or a side-view mirror; they come in hard, right into the front end of a cancer cell and destroy its DNA. In fact, one of the advantages of proton therapy over standard x-rays is that the proton beam’s radiation so thoroughly ravages the tumor’s DNA that its ordinary repair mechanisms are completely ineffective.
It is important to understand that the proton therapy beam is a safe form of treatment because its energy is very carefully controlled. If you trained the much more powerful proton beam from the large hadron collider on a brain tumor in a patient, it would turn that whole patient’s head into something called quark-gluon plasma, which would, for a whole bunch of reasons, be very awkward to have to explain to a jury in the event of a malpractice suit. The energy of the LHC beam is measured in tera electron volts, or a million times the energy used in medical proton beam treatments.
Prometheus gave us the gift of fire a long time ago; but, for better and for worse, we’ve now found a way, on our own, to play with nuclear matches. We humans can now confidently harness the enormous amounts of energy stored in atoms to answer deep questions about the universe, to create unimaginably powerful bombs or to shred the molecular machinery of a cancer cell.
Patients treated with proton therapy will include children, who are at greatest risk from the long-term effects of traditional radiation, and adults with a variety of localized cancers that haven’t yet spread. To date, the largest population of patients undergoing proton treatment are those with prostate cancer, a disease for which there are already a bewildering variety of alternative treatments. However, proton beam irradiation has major advantages over other forms of prostate treatment: It doesn’t require surgery and it can potentially avoid the worst side effects of traditional x-ray-based radiation and surgery, such as impotence and incontinence, by sparing the nerves, bladder and rectum around the prostate.
Proton beam therapy can be characterized as a medical tour de force, in which the treatment is delivered like a deus ex machina. The patient walks into a room, lies down on a bed, and, for the few minutes he’s there, all of this magical stuff happens around him and to him, painlessly, silently, perhaps while he listens to his iPod. Then he gets up and leaves after paying his co-pay. Although technically an outpatient treatment, it exemplifies the kind of scientifically sophisticated methods that advanced medical centers in the United States, Europe and Asia are deploying in next-generation facilities that are definitely not your father’s hospital, and not my father’s either.
William Hanson is a professor of anesthesiology and critical care at the School of Medicine, and director of surgical intensive care at the Hospital of the University of Pennsylvania. Excerpted from William Hanson, “The Edge of Medicine: The Technology That Will Change Our Lives,” 2008, Palgrave Macmillan. Reproduced with permission of Palgrave Macmillan.
SIDEBAR
Countdown to the First Proton-Beam Patient
One year and one month after workers lowered the 220-ton proton cyclotron into the construction site of the Roberts Proton Therapy Center, the building above it has come into being. But it will be just a while longer before anyone can book an appointment. In addition to the parade of federal inspectors who have to sign off on it, the equipment is in the midst of tests and simulations that will occupy several months.
Those will run the gamut from one end to the other of an astoundingly complicated pipe. “First, you turn on the cyclotron to see if it makes protons,” says Susan Phillips, vice president for public affairs at Penn Medicine. “We know it does that, because that test has already happened. Then you test the magnets that shape the beam to various treatment rooms. Then you have to test what comes out of the patient gantry. It’s literally thousands of calculations. And on top of that, there’s a tremendous amount of software and computer programming.”
After that, it’s a case of rinse, wash, repeat. “Each treatment room comes online by itself,” adds Phillips. “So you have to do it five times.
If everything goes according to plan, protons will start zapping tumors this November.
SIDEBAR
The Oldest Tool in Medicine
My father’s hands were large, warm, blunt-tipped and always well manicured. He was an internist and used his hands as diagnostic tools. His fingers probed his patient’s neck, abdomen, armpits and groin—the soft underbelly as it were—searching for enlarged nodes or organs. These are private parts and a patient is most comfortable when the examiner’s hands are warm, clean, well groomed and competent. My father knew this. He was reserved, and many people described him after his death as “a gentleman.” He shook my hand, in what must have been a very proud moment, as I was about to be inducted into the Philadelphia College of Physicians, an old honorary society dating back to the 1700s of which he was a member. He said to me quietly, “Your hands are warm, you’re not nervous. That’s good.”
As a child, with fascination I used to watch him trim, file and clean his nails. He even applied something called cuticle wax during one period, which was not, I think, an expression of personal vanity, because he wasn’t that way. It was done more in the line of a master craftsman’s maintenance of his tools, much as the carpenter would sharpen his planes, or a butcher hone his knives to a razor’s edge, with a drop of oil on a whetstone.
Physicians are taught to inspect, palpate, percuss and auscultate, or look, touch, tap and listen, and as a routine stage in the physical examination of a patient, my father would pass his hand under a patient’s garment to feel the character and location of the beat of the heart within the rib-cage. In order to feel the apex of a woman’s heart, he would have slid his right hand under her left breast, exactly as I later learned to do.
He touched with the tips and sides of his right index and third fingers—even a left-handed physician, as he was, examines the patient from the right side because that’s the most natural way to feel the heart. When percussing, he used the middle finger of his dominant left hand to strike the last knuckle of the middle finger of his right over the patient’s chest and stomach, acquiring information from the resulting sounds. I use my right hand to strike my left because I’m right-handed. The striking finger is called the plexor and the struck finger is the pleximeter. Done skillfully, percussive examination can tell the practitioner a lot about the character of the tissues and organs that lie beneath. A stomach full of gas sounds like a kettledrum. A healthy lung has a distant but still-hollow sound. The lung of a patient with emphysema sounds more resonant than normal, but a collapsed lung sounds dull. The liver sounds flat, more like muscle. An experienced examiner can map out the location and determine the character of major organs without need of an x-ray. It took me some time to become competent at percussion—the plexor finger must be kept rigid as the hand is snapped, rotating quickly through one of the wrist’s degrees of freedom toward the pleximeter finger of the opposite hand. The wrist stays loose and the finger firm. Unfortunately, physical examination is an increasingly lost art in the medical schools of today.
My father often used those same big blunt, skilled hands to examine painful areas, such as the belly of a patient with appendicitis or an inflamed gall bladder. Here, a doctor must strike a balance between touching firmly enough to gather necessary information and gently enough to minimize discomfort. A good physician improves the calibration of his hands as he goes through his career, touching, assessing, integrating and touching again.
All animals use touch to explore their world, and touch may be the most primitive sense. Parents feed, carry, teach and discipline their offspring using various modes of touch. Touch is important during courtship and coupling. Primates groom one another with their hands to reduce stress, win favors, avoid confrontation and establish or reinforce social hierarchy. We humans run to be touched by our parents, we hold hands, we go to barbers, manicurists and masseuses. As far as I know, however, humans are the only species that touch one another to diagnose and treat disease.
William Hanson is a professor of anesthesiology and critical care at the School of Medicine, and director of surgical intensive care at the Hospital of the University of Pennsylvania. Excerpted from William Hanson, “The Edge of Medicine: The Technology That Will Change Our Lives,” 2008, Palgrave Macmillan. Reproduced with permission of Palgrave Macmillan.