Blood Pressure by the Clock
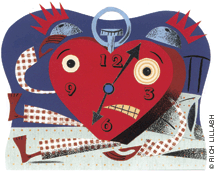
Doctors have known for years that heart attacks and strokes come most frequently in the morning, when blood pressure also tends to peak, but they haven’t been entirely sure why. On one hand, many body functions fluctuate in a circadian rhythm, suggesting that our molecular clocks may be responsible. On the other, the stress of waking up and hustling to work may be what makes the beginning of the day a risky time. Now researchers at the School of Medicine say both explanations are right, and they’ve found a gene that links them—and which might one day lead to medical strategies that could reduce the morning peril.
In a study published in the Proceedings of the National Academy of Sciences in February, the researchers found that blood pressure in mice fluctuates throughout the 24-hour cycle in tandem with catecholamines, the “fight or flight” hormones that govern stress responses. Furthermore, when the mice were subjected to environmental stresses, the degree to which catecholamines and blood pressure spiked in response depended on the time of day.
“Lo and behold, the biggest rise you get corresponds to the mouse version of the early morning hours for humans,” says senior author Dr. Garret FitzGerald, the Elmer Bobst Professor of Pharmacology and director of Penn’s Institute for Translational Medicine and Therapeutics.
Things got interesting when the researchers looked at mice in which a “molecular clock gene” called Bmal1 had been deleted. The jumps in blood pressure and catecholamines fell off significantly, and no longer varied with the time of day. Evidently, this clock gene plays a major role in governing stress responses. Since it’s found in humans as well, that opens the possibility—still distant—of using a specially targeted drug to alter its function in advantageous ways.
“You could [potentially] phase-delay the peak of blood pressure away from the time of day when you are most subject to stresses,” FitzGerald speculates.
Since catecholamines also influence the severity of asthma and depression—both of which also tend to be worse in the early morning—the molecular clock’s newfound role in governing these hormones might eventually have even broader therapeutic ramifications.
Single-Parent Stem Cells
Nearly a decade after the first line of human embryonic stem cells was produced in a petri dish, it’s hard to find an issue more hotly contested than the moral status of embryos. But a recent finding by Penn biologists may substantially change the debate. In an experiment published in a February issue of Genes & Development, researchers from Penn’s Center for Animal Transgenesis and Germ Cell Research proved that stem cells derived from single-parent mouse embryos—embryos in which the genetic material of sperm and eggs had never met—can regenerate an adult organ.
Scientists have known that by chemically prompting an unfertilized egg to begin dividing, even though it contains only the mother’s genetic material, it is possible to grow an early-stage embryo from which the highly versatile pluripotent stem cells can be derived. (A single-father embryo can be created by first replacing the egg’s genetic materials with that of two sperm from the same male.)
Dr. John McLaughlin, assistant professor in reproductive physiology, research associate Sigrid Eckardt, and research specialist Adrian Leu, collaborating with scientists from several other institutions, used both varieties of single-parent stem cells to generate fetal liver cells that they injected into sick mice.
“The mice were lethally irradiated, which means they would normally die in two weeks,” McLaughlin says. “By reconstituting them with these cells grown in vitro, they survive that period and actually live for several months.”
Some of the mice actually thrived for more than a year as the stem cells regenerated the damaged blood tissue. What’s more, says McLaughlin, “we can also take bone marrow from those mice and transplant it into other mice, and those stem cells will regenerate the bone marrow in that mouse.”
This experiment is the first to demonstrate that single-parent embryos have therapeutic value, and it comes as somewhat of a surprise. Although some plants, insects, and animals have the ability to produce offspring from unfertilized eggs—last year a Komodo dragon made headlines for such a “virgin birth”—mammalian embryos require chromosomes from two parents to develop into viable fetuses. But while single-parent cells lack the genetic equipment necessary for normal fetal development, evidently they can produce adult tissue efficiently and with no observed defects.
If the process can be made to work with other types of tissue and in humans, both of which McLaughlin hopes to explore in future experiments, it could expand the potential of regenerative medicine while sidestepping some ethical qualms. A patient undergoing in-vitro fertilization who had more eggs than she needed to produce a fertilized embryo, for example, could allow the unfertilized extras to be tapped for stem-cell production without conception ever taking place.
“They would never see a sperm,” McLaughlin says.
Why We Sniff
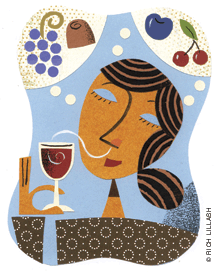
Wine aficionados are trained to plunge their noses into the bulb of a glass and sniff extra hard. While the casual sipper might find this audible snuffle pretentious or silly, a new study shows that when it comes to detecting faint odors, it pays to inhale with vigor. In an experiment on mouse olfactory neurons published in the March issue of Nature Neuroscience, assistant professor of neuroscience Minghong Ma found that a forceful rush of air can amplify the effect of a weak smell.
Most sensory systems in mammals are specialized to detect a single kind of stimulus. In the eye, for example, retinal neurons respond exclusively to light. It has long been known that olfactory neurons respond to chemical odor molecules, but Ma discovered that when subjected to pressurized flows of odorless air, they send a similar cascade of signals toward the brain—a chain reaction apparently governed by a messenger molecule called cAMP. To investigate what was happening on a cellular level, Ma and her co-authors peeled off the smell-sensing epithelial tissue from the nasal passages of mice, whose olfactory system is broadly similar to that of humans, and wired it with electrodes to record the rate of neuron firing. They found that when an odor was delivered at a standard concentration but different pressures, neurons fired more frequently as the pressure rose. In other words, individual cells seem to perk up when cued by forceful inhalation.
“When an animal encounters a very weak odor stimulus and does a powerful sniff, it does two things,” says Ma. “One thing is to pull in more air, which potentially will make the odor more concentrated in the nose. But the second is that now for individual cells, even at the same concentration of the odor, the chance for firing is higher. [That means] you can send [more] information to the brain with a high-pressure sniff.”
The sensitivity of olfactory neurons to airflows may also be a mechanism that allows the brain to synchronize odor detection with the natural rhythm of breathing. After all, perceiving smells when we inhale is more critical to assessing our surroundings than detecting odor molecules on their way out. —T.P.